Understanding the Basics of Germanium as a Semiconductor
In the realm of semiconductors, germanium (Ge) plays a starring role due to its distinctive properties suited for numerous applications. The inherent conductivity germanium possesses can be escalated dramatically by doping – an intricate process that involves infusing dopants or impurities into the germanium wafer to modify its electrical traits. The type and concentration level of the dopant utilized profoundly impact the semiconductor’s characteristics.
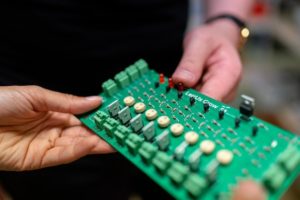
Two principal types of doping are recognized: n-type and p-type. N-type doping introduces pentavalent atoms into Ge’s lattice structure — atoms with five valence electrons, one more than what germanium inherently has. This extra electron turns into a carrier, enhancing conductivity within the semiconductor through facilitating better electron flow. Ion implantation is often employed as an effective technique for n-type doping because it provides precise control over both dopant concentration and depth distribution.
Yet, merely injecting dopants using ion implantation doesn’t automatically boost conductivity – it necessitates another step termed annealing or dopant activation; this heat treatment drives diffusion while also redistributing ions throughout the wafer and rectifying any flaws caused by ion bombardment during implantation procedure.
Henceforth, mastering how to effectively dope germanium requires expert knowledge in both techniques: ion implantation for accurate positioning of desired elements and subsequent annealing procedures for optimal activation and dispersion of these elements within our silicon-based semiconductor structure.
The Significance of Doping in Semiconductor Properties
As we delve into the intricate process of semiconductor fabrication, one stumbles upon a pivotal maneuver known as ‘semiconductor doping’. It is an intriguing method that involves weaving impurity atoms into the very fabric of an intrinsic semiconductor – silicon or germanium may serve as examples. The objective? To bring about a transformation in its properties; to enhance its conductivity by escalating carrier concentration. In this mystical dance of particles, these impurities come forth as dopants and either bestow free electrons (n-type doping) or graciously accept them (p-type doping), thereby manipulating the charge transport mechanism within our protagonist – the semiconductor.
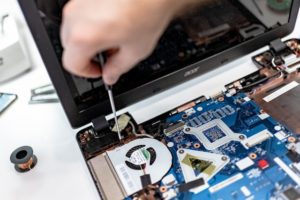
The role played by our dopant atom is akin to an actor donning different roles depending on n-type or p-type doping requirements. Consider germanium wafers for instance, doped with group V elements such as phosphorus or arsenic possessing five valence electrons. Four out of these five electrons form bonds with neighboring Ge atoms creating a lattice structure while one electron remains untethered and contributes to electrical conduction; thus birthing an n-type semiconductor. However, if germanium flirts with group III elements like boron or gallium armed with three valence electrons, they form incomplete bonds resulting in creation of ‘holes’ which act as carriers in p-type semiconductors.
Nevertheless, attaining pinpoint control over carrier concentration through diffusion-based stratagems presents itself quite challenging due to inherent complications related to defects in crystal lattice and variability in ion distribution across wafer thicknesses. As depicted vividly in figure 3, minor variations can wield significant influence on device performance making it imperative for manufacturers to maintain stringent controls during the entire process flow—from initial selection and preparation of silicon or germanium wafers all through their subsequent processing stages—to ensure consistent results from each batch produced.
Unpacking the N-type and P-type Doping in Germanium
The intriguing art of doping germanium, a member of the fourth group in the periodic table, consists of introducing foreign atoms into its natural structure with the intention to alter its electrical properties. We can divide this practice into two categories: n-type and p-type.
In crafting an n-type semiconductor, we summon an element from the fifth group as our choice dopant. This action results in infusing an additional electron – acting as a charge carrier – into germanium’s conduction band, causing an increase in carrier concentration that subsequently boosts conductivity.
By contrast, when sculpting a p-type semiconductor comes on stage, we pick up a player from group three to replace some germanium actors within their crystallographic lattice positions – defined as substitutional sites – which gives birth to ‘holes’ or vacancies playing their part as positive charge carriers. The method employed can range; however one frequently adopted technique harnesses pulsed laser anneal for diffusing dopants onto thin film stages such as silicon wafers. It is critical to underscore that this process influences not only resistivity but also leaves significant imprints on optical properties thus making it particularly beneficial for solar cell applications.
The wisdom gleaned from density functional theory provides insights at molecular level aiding us comprehend these procedures and predict device performance with increased precision. Figure 3 paints how varying degrees of dopant saturation affect both n- type and p- type semiconductors’ traits under diverse scenarios. However, maintaining exact control over these parameters becomes crucial since excessive concentrations might trigger unintended effects like diminished mobility due to heightened scattering events among carriers or even induce changes in material phase shifts. Therefore striking harmony between all these variables stands paramount within applied physics studies aimed at optimizing semiconductors based on Germanium.
Exploring the Role of Dopant Atoms and Ions in Germanium
The essential function of dopant atoms and ions in modifying the properties of germanium, an intrinsic semiconductor material, is undeniable. A process named doping introduces these impurities into the semiconductor, leading to a significant amplification of its electrical conductivity. This operation can be accomplished via methods such as ion implantation or chemical doping. In particular, there has been extensive research on doped Ge (germanium) using scanning electron microscope techniques to delve deeper into understanding the chemistry involved.
Depending on which dopant atom is used will yield either n-type or p-type semiconductor properties. For instance, implementation of n-type doping incorporates pentavalent elements like phosphorus or arsenic with five valence electrons. Conversely, p-type doping employs trivalent elements such as boron possessing three valence electrons for achieving specific outcomes. These dopants provide free carriers enhancing conductivity within the doped semiconductor system – a pivotal element affecting silicon germanium and organic semiconductors systems’ performance.
Moreover, it’s vital to comprehend how varying levels of doping impact germanium’s characteristics for optimizing its usage in assorted applications from solar cells to transistors. High-resolution imaging techniques offer intriguing insights about dopant activation within germanium nanowires and wafers – hence furnishing valuable data regarding their potential applications in advanced electronics industry sectors like energy harvesting devices and sensors among others . It’s also imperative that due diligence is exercised when selecting an appropriate dopant precursor during ion implantation processes to ensure optimal control over both dope level and distribution across the entire target area – thus facilitating efficient utilization of these versatile materials on a larger scale.
Enhancing Electrical Conductivity in Germanium through Doping
The endeavor of infusing a semiconductor such as germanium with dopants is executed via methodologies like diffusion or ion implantation. This intricate operation involves the introduction of impurities, known colloquially as dopants, into the pristine layer of germanium. The intent here is to alter its inherent properties and augment its electrical conductivity.
The effect that doping has on semiconductors like silicon or germanium (both partakers in group IV semiconductors) hinges heavily upon the variety of dopant used and their population within the given material.
Through meticulous regulation throughout this process, one can achieve an escalated concentration of desirable dopant. Take for example figure 3, which illustrates how heightened levels of doping can lead to superior carrier mobilities in both p-type and n-type germanium films. It’s worth noting at this juncture that while n-type films are typically doped with donor atoms such as phosphorus or arsenic (which donate free electrons), p-type films utilize acceptor atoms like boron or gallium (which fabricate holes). As a result, depending on whether an electron-rich (n-type) or hole-abundant (p-type) environment is necessitated for device functionality, different breeds of dopants are picked out from the lineup.
The grand finale in enhancing electrical conductivity in Ge using these techniques involves annealing facilitated by high temperatures. Annealing plays dual roles; firstly it mends any harm inflicted during the doping phase; secondly it enables redistribution of introduced impurities thereby ensuring uniformity across the material structure’s landscape. The extent to which these objectives are accomplished can be determined through various mathematical models that take into account parameters such as temperature configuration and time duration during annealing among other variables.
Therefore grasping how each phase influences overall performance becomes paramount when working towards optimizing conditions under which a doping mechanism should be carried out using Germanium semiconductors.
Comments are closed.